An ecological definition of health through the stress response
May 2021
by Dr. Sarah Aliko PhD
This piece of work aims to detail why the Urban Health Council focuses on the stress-response-system.
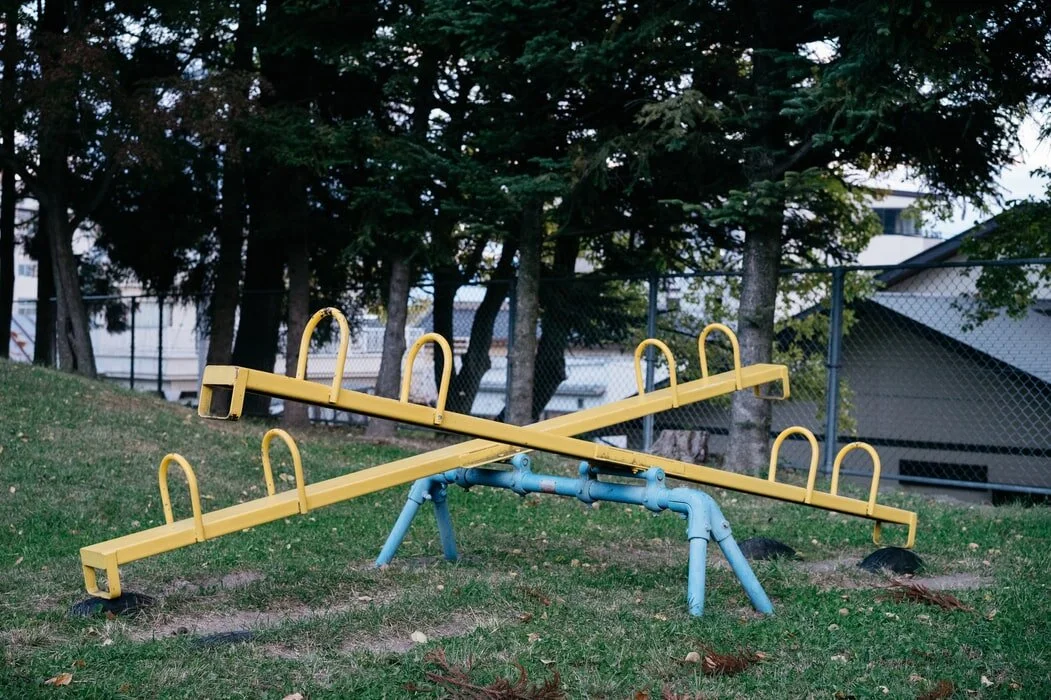
“A prolonged dysregulated condition of allostasis often results in an extreme state for the body, called allostatic overload. Differently from allostasis, allostatic overload is more chronic and intense in nature, and reflects the transition from normal wear-and-tear caused by daily demands, to a significant disruption of health, such as onset of diabetes or obesity”
Stressors & Stress Response
A stressor is defined as a novel threatening environmental agent that alters the baseline human biological system in either of two ways: bringing the system to an unstable biological state, or slowing down the system’s internal response so that it cannot reach equilibrium1. For clarity, environmental stressors can be both internal and external, such as infections and emotional stressors, or extreme weather conditions and trauma respectively 1,2.
In order to provide an adequate response to stressors, the body engages the stress response, a cascade of physiological reactions that act at two different time frames.
Firstly, a short-term response, also identified as ‘fight or flight’, allows the body to respond immediately to life-threatening events. This is achieved via a complex communication between the brain and endocrine, cardiovascular, immune and digestive systems2. Secondly, a long-term response occurs when the stressor persists for a significant amount of time, often preventing the body from returning to baseline biological parameters, thus posing a serious risk to health2.
In this frame, we define two types of stressors: an acute stressor is one that lasts for minutes or hours and results in the short-term stress response; a chronic stressor is instead one that persists for days or more, leading to the long-term stress response3.
The biological pathways involved in the stress response fall into two categories, namely the hypothalamic-pituitary-adrenal (HPA) axis and the autonomic nervous system (ANS). The malfunctioning of the two pathways is a leading cause of many pathologies, such as autoimmune diseases3-6. Although both play an important role in responding to stressors, the HPA-axis will be the main focus of this review, given that it is more widely studied and thus there is more evidence for its mechanisms. This pathway is based on a communication between the nervous and endocrine (hormones) systems, whereby glucocorticoids play a significant role as regulators6.
Importantly, the HPA-axis is tightly controlled by negative-feedback loops, which ensure that hormonal levels remain within a healthy range for the body7.
Homeostasis vs Allostasis
Homeostasis is a long-debated concept in the field of neuroscience and immunology. The term was originally coined by Cannon, who defined it as “a condition - a condition which may vary, but which is relatively constant”8,9,10.
Recently, McEwen has provided a more comprehensive definition of homeostasis, explaining it as the return to a predetermined set of physiological conditions of equilibrium of the body, following stress2. Of note is the fact that both authors refer to homeostasis as a return to equilibrium, rather than an immobile state of the system.
The semantic debate over homeostasis has intensified since the introduction of the concept of allostasis by Sterling and Eyers. They claimed that the term homeostasis is misleading, and that instead the body must respond to environmental stressors by altering all the baseline parameters through a process of allostasis11.
In their original article, homeostasis was interpreted as a state of static equilibrium, rather than a process that allowed a return to baseline parameters, as postulated by Cannon12. In their description, allostasis encompasses both baseline physiological parameters necessary for survival, and the dynamic mechanisms needed to maintain them over time.
In order to help solve this historical debate, McEwen offered a unifying model of homeostasis and allostasis, whereby allostasis is complementary to homeostasis and is the process that maintains homeostasis through dynamically-changing physiological parameters (eg. blood pressure and heart rate), to respond to environmental demands2.
In his model, homeostasis refers to the stability of core tissue parameters, such as pH and temperature, while allostasis dynamically modifies pericellular parameters (eg. blood pressure and oxygen) to maintain the core parameters stable (homeostasis) over time13.
Importantly, he explained that, differently from homeostasis that is the basis of survival, allostasis is a process that allows adaptation over time2,14. The two terms have been interchangeably used in the literature, even to this day12,14. In this review we will use the following definitions of homeostasis and allostasis:
- Homeostasis is the return to predetermined healthy baseline biological parameters (both core tissue and pericellular), such as pH of 7, body temperature of ~37℃, blood oxygen at 99% etc. Homeostasis is important for survival
- Allostasis refers to processes that respond to environmental (internal/external) challenges by altering pericellular physiological parameters, so as to maintain homeostasis over time. Allostasis is important for adaptation
The body enters an allostatic state when allostasis has been active for a long period of time, and thus the mediators are deviated from their normal levels to a new set point, that can be lower or higher than the normal one 14,15. Being in an allostatic state comes at a cost to the body, especially when responding to chronic stressors that prevent the stress response from turning off2. Over time, exposure to multiple stressors (eg. demands of daily routines, injury, disease, age etc) lead to changes in the mediator, and ultimately, physiological parameter levels, culminating in a cumulative effect on the stress response called allostatic load2,14. Allostatic load is reached through one of four possible responses:
- repeated novel stressors cause spikes in mediator levels;
- failure to adapt to the same stressor;
- failure to turn off the stress response;
- overactive responses 2,16,17.
The physical repercussions of allostatic load manifest in a general sense of being ‘stressed out’, resulting in fatigue, anger and frustration among others 2,5,16.
A prolonged dysregulated condition of allostasis often results in an extreme state for the body, called allostatic overload (Fig.1)14.
Differently from allostatic load, allostatic overload is more chronic and intense in nature, and reflects the transition from normal wear-and-tear caused by daily demands, to a significant disruption of health, such as onset of diabetes or obesity2,18.
Figure 1. Homeostasis, allostasis and dysregulated response. The diagram shows two scenarios: 1) a normal response where the body is subject to acute stressors and allostasis is able to restore homeostasis; 2) a dysregulated response where repeated acute or chronic stressors cause a prolonged allostatic state, leading to allostatic load and overload.
Although the terms allostatic load and allostatic overload are often mentioned in biomedical literature, there are no formal quantitative definitions for the two concepts.
In particular, allostatic overload is used to express extreme dysregulation of the stress response 19, but no “threshold” for when the body enters this phase has been proposed. Supposedly, this is because the circumstances in which allostatic overload is triggered vary significantly from one individual to the next, and a stressor may not result in allostatic overload in all cases 20.
A further challenge with setting thresholds is that there is lack of agreement over what to measure to determine allostatic load/allostatic overload: are particular mediators and levels (eg. cortisol and other hormones) enough, or should we also consider contributing variables (eg. age, gender, diet, habitat, health behaviours)? 2, 14
The issue with these arbitrary definitions is that many studies then identify genetic makeup as the leading cause for complex diseases such as depression, rather than dysregulation from chronic exposure to stressors 21.
Below we clarify the timeline of events from homeostasis to allostatic overload along with likely outcomes of allostatic load and allostatic overload. Fig.2 indicates that allostatic overload is the moment when disease onset begins, where no return to homeostasis is possible and a new “baseline” for the body has been created by shifting the optimal baseline homeostasis parameters (eg. blood pressure etc). Of note is the fact that the new baseline under AOL does not equate homeostasis, instead is an unhealthy dysregulated baseline caused by persistent exposure to stress.
It could be argued that allostatic load happens even when humans adapt to harsh environments (eg. really cold climates) and, as a result, the baseline parameters may change. The important distinction is that the engagement of allostatic load in individuals moving to the North Pole, eventually changing their baseline parameters, is a natural phenomenon of adaptation and evolution. Instead, exposure to high air pollution is an artificial outcome of human activity that results in unadapted baseline parameters and disease. Nature should determine what the human body is subjected to, not human activity. This difference can be better explained by looking at wildlife species. Extinction of species is an event driven by natural selection, but in recent decades widespread mass extinctions have been taking place as a result of human activity: this unnatural selection is being applied to communities of our society.
Figure 2. Timeline of allostasis. The diagram shows the short-term (hours/days) action of allostasis to restore homeostasis; allostatic load happens when allostasis is engaged for more prolonged periods (months/ few years) causing feelings commonly termed as stress or low immune defenses; finally chronic and persistent exposure to stressors and in a state of allostatic load lead to overload and thus disease onset.
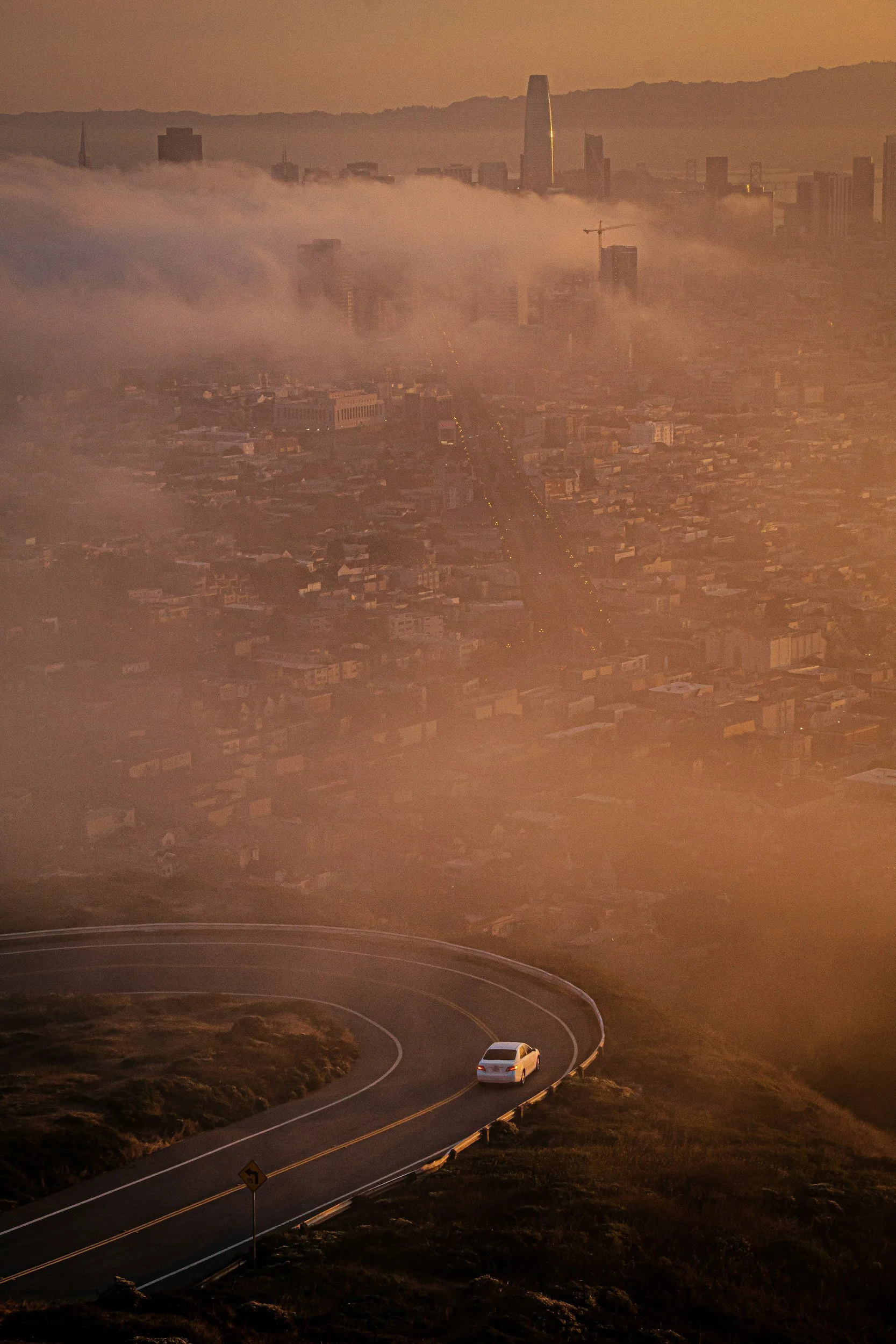
“The important distinction is that the engagement of allostatic load in individuals moving to the North Pole, eventually changing their baseline parameters, is a natural phenomenon of adaptation and evolution. Instead, exposure to high air pollution is an artificial outcome of human activity that results in unadapted baseline parameters and disease. Nature should determine what the human body is subjected to, not human activity.”
Allostasis & Disease Biomarkers
As mentioned above, allostatic load and allostatic overload are states in which the system enters a dysregulated stress response. Non-communicable diseases have been shown to be associated with allostasic overload 18. For instance, the following disorders are linked:
- Cardiovascular diseases, such as coronary or ischemic heart disease and peripheral arterial disease
- Type II diabetes
- Muscoloskeletal disorders, such as chronic fatigue syndrome and fibromyalgia
- Cancers, such as breast and ovarian cancer
- Depression, anxiety and PTSD
Non-communicable diseases are diagnosed using either physiological biomarkers (eg. glucose levels, blood pressure etc) of the stress response or through clinical assessment questionnaires that are aimed at qualitatively estimating levels of psychosocial stress 18. The latter offers a more personalised assessment of an individual’s experience of stress and their resilience, and how these affect risk of developing disease 2, 18, 22. It is in this framework that the allostatic load index has been developed. It includes ten different biological parameters ranging from physiological to hormonal parameters, such as body mass index and cholesterol levels, that help in assessing the level of chronic stress of an individual 23.
The parameters included in the allostatic load index are divided into three scales: primary, secondary and tertiary.
- Primary parameters are physiological ones that modulate the basic stress response;
- secondary are the ones that develop as a cumulative effect of the primary biomarkers;
- tertiary are the disease biomarkers resulting from a chronic presence of secondary parameters 24.
Overall, the allostatic load index seems to predict mortality and physical decline better than traditional methods using individual biomarkers of disease 2.
Although advances in our understanding of allostatic load and the development of the allostatic load index have improved our prediction of disease risks, some issues still persist. Indeed, western medicine is founded on the principle of curing or alleviating symptoms of disease rather than aiming to prevent their onset. Future studies on allostatic overload should design methods to help early diagnosis and prevention of non-communicable diseases by looking at allostatic load indices.
The nature of allostatic load and allostatic overload, particularly their ability to permanently modify the baseline physiological parameters (homeostasis) of an individual, raises questions regarding the mechanisms behind developmental disorders such as autism.
If dysregulated stress response biomarkers were demonstrated to be transmitted from parent to offspring, this would shed new light on the origins of complex developmental disorders.

“…in order to better understand why certain communities are being disproportionately affected by the incidence of non-communicable diseases, it is important to consider the environmental and psychosocial stressors that the community experiences daily.”
Biological Inequity
Although the prevalence and incidence of non-communicable diseases has significantly increased in the last few decades across the western world, there are parts of society that are being disproportionately affected by them.
Epidemiological studies have attempted to explain these differences through genetic or epigenetic processes, which are hereditary or developed through the course of life. These imply that the individual is the sole agent that determines health outcomes 25, 26. Many such studies, however, investigate health in the sterile isolated settings of laboratories, without taking into consideration the day-to-day experiences of the person nor the environment they constantly interact with.
Indeed, exposure to stressful environments and events have been shown to dysregulate the stress response, often leading to disease onset. For instance, exposure to chronic stress was shown to be linked to higher risk of dementia as well as autoimmune diseases and mental health disorders 27, 28. Risk of disease also increases with higher numbers of stressors, which act in a synergistic manner to further and more powerfully disrupt the proper functioning of the stress response 4. As we previously discussed, stressors can derive from different sources, both psychological and environmental (eg. pollution).
Thus, in order to better understand why certain communities are being disproportionately affected by the incidence of non-communicable diseases, it is important to consider the environmental and psychosocial stressors that the community experiences daily. It is from these considerations that we propose a new term to describe the unequal distribution of stressors and their effects on health in our society: biological inequity.
We introduce a new term called biological inequity to describe the fact that different communities experience very different levels of stress - and as a result very different health outcomes - due to the systemic application of racism and classism. Chronic exposure of some communities to poverty, pollution, trauma and other stressors are drivers for biological inequity 29, 30. In this framework, it is not the individual’s genetic makeup that is the indicator of health, but the environment and daily experiences they are constrained to live in. That is not to say that genetics play no role in determining health. Rather, it means that biological inequity is a more encompassing explanation for differences in health outcomes. For instance, two women carrying the same gene for breast cancer but living in different environments will have different health outcomes: it is more likely that one living in polluted areas and experiencing daily stress or trauma will experience cancer onset, while the other living in a clean environment might not.
Moreover, the mechanisms of biological inequity are more unbiased than traditional epidemiological explanations of health. In fact, epidemiological research has often proposed that genetic distinctions between ethnicities are the driver of poor health outcomes for certain minority communities 31, 32, 33. Instead, biological inequity proposes that the systemic application of racism and classism forces certain communities to live in environments with higher stressor levels than normal, thus resulting in poor health outcomes.
In this framework, the epigenetic differences between ethnicities described in the literature can be explained through allostatic load/allostatic overload: a permanently dysregulated stress response due to the environment can cause epigenetic changes that are transmitted from parent to offspring, becoming hereditary 19, 28, 29. The inner workings of biological inequity can be summarised in Fig.3.
Figure 3. The stress response is at the intersection between environmental (pollution), psychosocial stressors and an individual’s sensitivity (eg. based on age etc). When racism and classism are applied they increase environmental and psychosocial stressors, disproportionately affecting the stress response. The results of a constantly dysregulated stress response affect the individual’s lifespan and can be detected at different life stages: from in utero to adulthood. These changes may lead to epigenetic effects that become hereditary. (Figure adapted from https://paperpile.com/c/iC1Gsa/gRhP)
Impact of Pollution on Health
There is a large consensus in the literature that meeting a threshold for air pollution levels is sufficient to guarantee health 34. Indeed, the World Health Organization (WHO) has set out specific thresholds for acceptable levels of pollution 34, which are used by governments across the world as golden standards. However, the human body is not naturally designed to live in continuously polluted environments 35.
Research on the effects to health of air pollution have shown that individuals had higher life expectancy and improved lung functioning when exposed to lower levels of pollution, whilst they suffer higher prevalence of neurodegenerative disorders at higher levels 36, 37.
Recently, we have conducted a study on the effects of air pollution produced from works in a brownfield site on a community in Southall, London. Healthcare and local authorities have argued that the levels of air pollution emitted by the construction site are within WHO standards, therefore do not pose a risk to health according to traditional definitions of health. Nevertheless, the community has experienced significant adverse health effects, reporting increased prevalence of asthma and other respiratory diseases.
Our investigation has indicated that because of the history and daily stressors encountered by the community, the WHO pollution standards are not applicable, and lower “standards” should be achieved to protect the community’s health 38. Indeed the community is mostly composed of ethnic minority individuals and is one of the most deprived in the UK (lowest 10%). These facts indicate that systemic racism has forced the community to live in a highly deprived area, likely causing a dysregulated stress response in many individuals. This dysregulation has made the community more susceptible to air pollution, meaning that “standard” WHO guidelines cannot be applied here.
Updating Definitions of Health
We propose an ecological definition of health that is based on the stress response and its ramifications. Health is not static nor restrained to the human body, but is the result of a constant and dynamic interaction between the person and the environment.
Healthcare in Western society is often simplified to a checklist of common symptoms to diagnose disease and standard treatments (often involving drugs) as cures. These overlook the differences in lived experience of individuals, and have a negative impact on deprived and ethnic minority communities.
Inadvertently, current healthcare models are exacerbating social divides by not acknowledging the disproportionate levels of stressors in certain communities.
Our new definition of health is in line with the WHO: they initially defined health as a state of well-being with absence of illness, later introducing a link to the HPA-axis, by framing health as the ability to restore homeostasis after stress 44, 45.
Although these definitions have long existed, they have been disregarded in favour of more biomedical approaches that use quantitative measures of disease biomarkers. We offer a revisitation of this interpretation of health, and offer further possibilities on how to apply it. Even though the WHO’s official definition is one that incorporates notions of allostasis, our health definition expands to ideas of equity.
We propose that health services assess a patient’s exposure to stressors on an individual basis and work to understand allostatic load and prevent allostatic overload, and therefore disease, rather than focus on reactive therapies after disease onset.
This should be achieved by communicating with local authorities and governmental bodies and developing plans for improving air quality, promoting healthy lifestyles and social cohesion and supporting communities, for example by reducing deprivation and creating better and more accessible urban spaces.
Importantly, these regeneration and public awareness plans should be fairly distributed based on needs: by this we do not simply mean equality, but equity. Taken together, these measures would not only prevent communities from being subjected to biological inequity, but would also increase community resilience, meaning the ability to strengthen and adapt to external challenges 46, 47, 48. Overall, acting on preventative measures rather than reactive ones, will reduce the incidence of non-communicable diseases and thus the burden on health and social services.
The Urban Health Council will focus on an ecological view of health through systems biology, neuroendocrinology and neuroscience. We term this umbrella of biology disciplines as ‘systems neurohealth’.
-
1. Oken, B. S., Chamine, I. & Wakeland, W. A systems approach to stress, stressors and resilience in humans. Behav. Brain Res. 282, 144–154 (2015).
2. McEwen, B., Nasveld, P., Palmer, M. & Anderson, R. Allostatic Load A Review of the Literature. (2012).
3. Won, E. & Kim, Y.-K. Stress, the Autonomic Nervous System, and the Immune-kynurenine Pathway in the Etiology of Depression. Curr. Neuropharmacol. 14, 665–673 (2016).
4. Schneiderman, N., Ironson, G. & Siegel, S. D. Stress and health: psychological, behavioral, and biological determinants. Annu. Rev. Clin. Psychol. 1, 607–628 (2005).
5. McEwen, B. S. Stressed or stressed out: what is the difference? J. Psychiatry Neurosci. 30, 315–318 (2005).
6. Smith, S. M. & Vale, W. W. The role of the hypothalamic-pituitary-adrenal axis in neuroendocrine responses to stress. Dialogues Clin. Neurosci. 8, 383–395 (2006).
7. Stephens, M. A. C. & Wand, G. Stress and the HPA axis: role of glucocorticoids in alcohol dependence. Alcohol Res. 34, 468–483 (2012).
8. Davies, K. J. A. Adaptive homeostasis. Mol. Aspects Med. 49, 1–7 (2016).
9. Chovatiya, R. & Medzhitov, R. Stress, inflammation, and defense of homeostasis. Mol. Cell 54, 281–288 (2014).
10. Cannon, W. B. ORGANIZATION FOR PHYSIOLOGICAL HOMEOSTASIS. Physiol. Rev. 9, 399–431 (1929).
11. Ramsay, D. S. & Woods, S. C. Clarifying the roles of homeostasis and allostasis in physiological regulation. Psychol. Rev. 121, 225–247 (2014).
12. Day, T. A. Defining stress as a prelude to mapping its neurocircuitry: no help from allostasis. Prog. Neuropsychopharmacol. Biol. Psychiatry 29, 1195–1200 (2005).
13. McEwen, B. S. & Stellar, E. Stress and the individual. Mechanisms leading to disease. Arch. Intern. Med. 153, 2093–2101 (1993).
14. McEwen, B. S. & Wingfield, J. C. What is in a name? Integrating homeostasis, allostasis and stress. Hormones and behavior vol. 57 105–111 (2010).
15. Ullmann, E. et al. From Allostatic Load to Allostatic State-An Endogenous Sympathetic Strategy to Deal With Chronic Anxiety and Stress? Front. Behav. Neurosci. 13, 47 (2019).
16. McEwen, B. S. Protective and damaging effects of stress mediators: central role of the brain. Dialogues Clin. Neurosci. 8, 367–381 (2006).
17. McEwen, B. S. & Seeman, T. Protective and damaging effects of mediators of stress. Elaborating and testing the concepts of allostasis and allostatic load. Ann. N. Y. Acad. Sci. 896, 30–47 (1999).
18. Guidi, J., Lucente, M., Sonino, N. & Fava, G. A. Allostatic Load and Its Impact on Health: A Systematic Review. Psychother. Psychosom. 1–17 (2020).
19. Thomson, E. M. Air Pollution, Stress, and Allostatic Load: Linking Systemic and Central Nervous System Impacts. J. Alzheimers. Dis. 69, 597–614 (2019).
20. McEwen, B. S. Allostasis and allostatic load: implications for neuropsychopharmacology. Neuropsychopharmacology 22, 108–124 (2000).
21. Binder, E. B. et al. Polymorphisms in FKBP5 are associated with increased recurrence of depressive episodes and rapid response to antidepressant treatment. Nat. Genet. 36, 1319–1325 (2004).
22. Fava, G. A. et al. Clinical characterization of allostatic overload. Psychoneuroendocrinology 108, 94–101 (2019).
23. Seeman, T. E., Singer, B. H., Rowe, J. W., Horwitz, R. I. & McEwen, B. S. Price of Adaptation—Allostatic Load and Its Health Consequences: MacArthur Studies of Successful Aging. Arch. Intern. Med. 157, 2259–2268 (1997).
24. McEwen, B. S. Biomarkers for assessing population and individual health and disease related to stress and adaptation. Metabolism 64, S2–S10 (2015).
25. Moosavi, A. & Motevalizadeh Ardekani, A. Role of Epigenetics in Biology and Human Diseases. Iran. Biomed. J. 20, 246–258 (2016).
26. Tzika, E., Dreker, T. & Imhof, A. Epigenetics and Metabolism in Health and Disease. Front. Genet. 9, 361 (2018).
27. Johansson, L. et al. Midlife psychological stress and risk of dementia: a 35-year longitudinal population study. Brain 133, 2217–2224 (2010).
28. Gold, P. W. The organization of the stress system and its dysregulation in depressive illness. Mol. Psychiatry 20, 32–47 (2015).
29. Aroke, E. N. et al. Could epigenetics help explain racial disparities in chronic pain? J. Pain Res. 12, 701–710 (2019).
30. Kim, P. et al. Effects of childhood poverty and chronic stress on emotion regulatory brain function in adulthood. Proc. Natl. Acad. Sci. U. S. A. 110, 18442–18447 (2013).
31. Barnes, L. L. Biomarkers for Alzheimer Dementia in Diverse Racial and Ethnic Minorities-A Public Health Priority. JAMA neurology vol. 76 251–253 (2019).
32. Suzuki, T., Israr, M. Z. & Salzano, A. Biomarkers in Cardiovascular Disease: The Dilemma of Racial Differences. Journal of the American Heart Association vol. 8 e014295 (2019).
33. Gijsberts, C. M. et al. The ethnicity-specific association of biomarkers with the angiographic severity of coronary artery disease. Neth. Heart J. 24, 188–198 (2016).
34. Ambient (outdoor) air pollution. https://www.who.int/news-room/fact-sheets/detail/ambient-(outdoor)-air-quality-and-health.
35. Barnett, A. G. It’s safe to say there is no safe level of air pollution. Aust. N. Z. J. Public Health 38, 407–408 (2014).
36. Kelly, F. J. & Fussell, J. C. Air pollution and public health: emerging hazards and improved understanding of risk. Environ. Geochem. Health 37, 631–649 (2015).
37. Ranft, U., Schikowski, T., Sugiri, D., Krutmann, J. & Krämer, U. Long-term exposure to traffic-related particulate matter impairs cognitive function in the elderly. Environ. Res. 109, 1004–1011 (2009).
38. Air Pollution and Health in Southall. https://static1.squarespace.com/static/57a5a729414fb58fa3e0e0a6/t/5ebd02d24fa06d4c4cd00b20/1589445337817/Southall+Report.pdf (2020).
39. Giddens 7th Edition. https://politybooks.com/giddens7/studentresource/summaries/Student_Summary_11.asp.
40. Mehta, N. Mind-body Dualism: A critique from a Health Perspective. Mens Sana Monogr. 9, 202–209 (2011).
41. Gendle, M. H. The Problem of Dualism in Modern Western Medicine. Mens Sana Monogr. 14, 141–151 (2016).
42. Lake, J. & Turner, M. S. Urgent Need for Improved Mental Health Care and a More Collaborative Model of Care. Perm. J. 21, 17–024 (2017).
43. Maragkoudakis, P. Cost of Non-Communicable Diseases in the EU. https://ec.europa.eu/jrc/en/health-knowledge-gateway/societal-impacts/costs (2020).
44. World Health Organization. The First ten years of the World Health Organization. (World Health Organization, 1958).
45. World Health Organization. Regional Office for Europe. Health promotion : a discussion document on the concept and principles : summary report of the Working Group on Concept and Principles of Health Promotion, Copenhagen, 9-13 July 1984. https://apps.who.int/iris/handle/10665/107835 (1984).
46. PHE. Community Resilience. https://www.phe.gov/Preparedness/planning/abc/Pages/community-resilience.aspx (2015).
47. Ozbay, F. et al. Social support and resilience to stress: from neurobiology to clinical practice. Psychiatry 4, 35–40 (2007).
48. McEwen, B. S. The untapped power of allostasis promoted by healthy lifestyles. World Psychiatry 19, 57–58 (2020).
49. Butt, E. W. et al. Global and regional trends in particulate air pollution and attributable health burden over the past 50 years. Environ. Res. Lett. 12, 104017 (2017).
50. Hong, C. et al. Impacts of climate change on future air quality and human health in China. Proc. Natl. Acad. Sci. U. S. A. 116, 17193–17200 (2019).
51. Alan Buis NASA’s Global Climate Change Website. A Degree of Concern: Why Global Temperatures Matter – Climate Change: Vital Signs of the Planet. https://climate.nasa.gov/news/2878/a-degree-of-concern-why-global-temperatures-matter/.
52. Mousavi, M. E., Irish, J. L., Frey, A. E., Olivera, F. & Edge, B. L. Global warming and hurricanes: the potential impact of hurricane intensification and sea level rise on coastal flooding. Clim. Change 104, 575–597 (2011).
53. White, C. & Nafilyan, V. Coronavirus (COVID-19) related deaths by ethnic group, England and Wales - Office for National Statistics. https://www.ons.gov.uk/peoplepopulationandcommunity/birthsdeathsandmarriages/deaths/articles/coronavirusrelateddeathsbyethnicgroupenglandandwales/2march2020to10april2020 (2020).